Platelet α-granule secretion is spatially regulated at the micro- and nanoscales
Lead Researcher: Yumiko Sakurai, MS

Platelet spreading beyond blue protein microdot boundaries is associated with increased green P-selectin expression in those specific regions of red unpermeabilized platelets (subfigure A). High levels of anti-P-selectin staining co-localize with regions of platelets that spread beyond the geometric boundaries of protein microdots and this effect inversely correlates with microdot size (subfigure B). Permeabilized platelets on adjacent protein microdots form bridging connections associated with high levels of P-selectin in those regions (subfigure C). Protein micropatterns with arrays of nanoscale “holes” reveals that P-selectin co-localizes with the patterned holes in permeabilized platelets (subfigure D).
Monitoring the “lifetime” of a thrombus over long timescales by leveraging a novel microvasculature-on-chip thrombosis resolution assay
Lead Researcher: Yongzhi Qiu, PhD

Treatment of thrombosis relies on prompt resolution of thrombi to restore blood flow to avoid ischemic injury. However, our understanding of the step-by-step process of how thromboses resolve remains limited due in large part to the lack of sufficient technologies. In addition, as thromboses require days to weeks to resolve, existing in vitro and in vivo systems cannot monitor this process, especially in the microvasculature where thrombi are difficult to visualize. As such, questions such as how do the cellular and biochemical composition of a clot change as it resolves and how do hemodynamics affect this process remain unanswered. This is particularly important in thromboinflammatory conditions such as autoimmune/inflammatory disorders in which patients are chronically at risk for microvascular thromboses but the pathophysiology and therefore optimal therapies remain unclear. Thus, a pressing need exists for an assay that assesses how microvascular thromboses resolve over long time scales, especially under thromboinflammatory conditions. In this project, we leverage the novel hydrogel-based microvasculature-on-chip system that we recently developed to monitor not only how microvascular thromboses form but, importantly, how they resolve over long timescales. Moreover, our system also enables the monitoring of how antithrombotic drugs and anticoagulants “work” in the context of existing inflammatory thrombi, which will provide insight into the pathophysiology as well as provide evidence for the use of different therapies.
Linking circulatory turbulence and sickle cell disease pathophysiology
Lead Researcher: Eudorah Vital

We have adopted a multi-well-plate vortex mixer paradigm to subject small volumes of sickle cell blood to turbulence with the advantage of assaying multiple cellular and temporal conditions. Traditional assays do not allow for the observation of the cellular processes that result from turbulent sRBC suspensions traveling through the vasculature. The contributions of this investigation will provide a better understanding of the dynamic conditions that cause sickle cell hemolysis. Thus, enabling the predictions of when and where it will occur in the vascular network and how to mitigate it. As the lifespan of SCD patients increases, these patients are at heightened risk of vascular conditions that can alter their physiologic flow regimes. This data can inform treatment guidelines for this population. Additionally, characterizing how turbulent sickle cell suspensions affect the endothelium can provide more insight into the mechanisms of endothelial dysfunction observed in SCD.
iCLOTS: open-source, artificial intelligence-enabled software for analyses of blood cells in microfluidic and microscopy-based assays
Lead Researcher: Meredith Fay, PhD

While microscopy-based cellular assays, including microfluidics, have significantly advanced over the last several decades, there has not been concurrent development of widely-accessible techniques to analyze time-dependent microscopy data incorporating phenomena such as fluid flow and dynamic cell adhesion. As such, experimentalists typically rely on error-prone and time-consuming manual analysis, resulting in lost resolution and missed opportunities for innovative metrics. We present a user-adaptable toolkit packaged into the open-source, standalone Interactive Cellular assay Labeled Observation and Tracking Software (iCLOTS). We benchmark cell adhesion, single-cell tracking, velocity profile, and multiscale microfluidic-centric applications with blood samples, the prototypical biofluid specimen. Moreover, machine learning algorithms characterize previously imperceptible data groupings from numerical outputs. Free to download/use, iCLOTS addresses a need for a field stymied by a lack of analytical tools for innovative, physiologically-relevant assays of any design, democratizing use of well-validated algorithms for all end-user biomedical researchers who would benefit from advanced computational methods.
Building the foundation of health-related knowledge via near-peer education for children with sickle cell disease
Lead Researcher: Elaissa Hardy, PhD

Health education for children with chronic illnesses (i.e., sickle cell disease [SCD]) has focused on educating adult caregivers with minimal consideration to educating the pediatric patients. We introduce a pediatric-focused educational paradigm, health-related knowledge (HRK), teaching pediatric patients developmentally appropriate general health literacy, and disease-specific knowledge. Using science, technology, engineering, and mathematics (STEM) education concepts, pediatric-specific HRK interactive activities address educational gaps: (a) general STEM education; and (b) general health and disease-specific knowledge to improve clinical outcomes. Total 144 pediatric SCD patients completed HRK activities, revealing overwhelmingly positive feedback (87%). Seventy-five percent of participants in 6th grade and above demonstrated thorough understanding of the STEM/HRK topics taught.
Pathologic mechanobiological interactions between red blood cells and endothelial cells directly induce vasculopathy in iron deficiency anemia
Lead Researcher: Christina Caruso, MD

Sickle cell disease (SCD), while caused by a single point mutation in the hemoglobin gene, is a systemic, vasculopathic disease in which endothelial cells are dysfunctional and inflamed. There remains much to learn about SCD vasculopathy, particularly regarding red blood cell-endothelial interactions, and my work aims to address this gap via a multidisciplinary approach including in silico, in vitro, and in vivo modalities. Additionally, my research has expanded beyond SCD to study red blood cell-endothelial interactions and endothelial dysfunction in other hematologic disorders and disease states, including iron deficiency anemia and diabetes.
Redefining Hyperviscosity in Acute Leukemia: Implications for Red Cell Transfusions
Lead Researcher: Jamie Oakley Musick, MD

Hyperleukocytosis, most commonly defined as a white blood cell (WBC) count greater than 100,000/μL, is an emergency in acute leukemia, possibly resulting in life-threatening microvascular obstruction, or leukostasis, leading to neurologic (CNS hemorrhage, thrombosis) or pulmonary (respiratory distress, hypoxia) complications. The underlying mechanisms remain poorly understood and are canonically attributed to blood hyperviscosity secondary to high WBC count and abnormal biophysical properties of leukemia cells themselves (leukemia immunophenotype, increased cell size, adhesion, and stiffness). Leukapheresis is a commonly-used therapy for rapid cytoreduction in symptomatic patients, but the procedure carries risk and existing guidelines are supported by scant evidence. Interestingly, despite hematocrit(Hct)/hemoglobin(Hgb) levels being major drivers of blood viscosity due to the high prevalence of circulating red cells (RBCs), how Hct/Hgb mediates hyperviscosity in acute leukemia is unknown. This is clinically important as Hct/Hgb often decrease as leukemic cell counts rise, and acute leukemia patients with anemia are often transfused. While sickle cell disease guidelines advise using a target post transfusion Hct of 30% to minimize iatrogenic hyperviscosity and its morbid complications, no guidelines have been established for acute leukemia. As such, can RBC transfusion actually increase leukostasis risk in acute leukemia?
Introducing a Novel Biophysical Platelet Function Panel to Investigate Disorders of Primary Hemostasis and Bleeding of Unknown Cause
Lead Researcher: Sally Azer, MD

A subset of patients with chronic bleeding remain undiagnosed even after extensive diagnostic evaluation are labeled as “bleeding of unknown cause” (BUC). The key barrier to treating these patients is that they have a clinical bleeding tendency in the presence of normal diagnostic tests, and optimal methods for monitoring and treating patients with BUC remain unknown. While patients with BUC have symptoms of a primary hemostatic disorder, there is no diagnostic test or biomarker that can accurately identify which patients are at risk for bleeding such as those with mild Von Willebrand Disease (VWD) which comprise a broad spectrum of patients with varying degrees of bleeding. In order to fill this diagnostic gap in disorders of primary hemostasis, there is a clinical need for more assays of platelet function. To that end, we have engineered multiple new biophysical assays to assess disorders of primary hemostasis and apply this panel of platelet function testing to potentially define new bleeding disorders, characterize platelet phenotypes in patients with BUC, and refine the definition of mild VWD. Our panel of platelet function tests collectively enables us to simultaneously assess different facets of primary hemostasis from the microscopic level of single-platelet physiology to hemostatic plug formation, thereby capturing various aspects of platelet function with a single blood sample. Our platelet function panel ranges from platelet adhesion and bulk clot contraction assays to spatially-regulated platelet granule secretion assay, single-platelet contraction cytometry, microfluidics, and a microengineered vascularized bleeding model. As such, we are leveraging these biophysical assays to correlate platelet function with bleeding phenotype severity and establish the dynamic range of this diagnostic panel. Ongoing research is being conducted using our comprehensive platelet function panel to investigate platelet properties in BUC and mild VWD and correlate these biophysics with bleeding phenotypes. Using this approach we aim to provide novel diagnostic testing with clinical relevance for disorders that have been incompletely characterized until now.
Heterogeneity in Neutrophils and Platelets
Lead Researcher: Hyoann Choi
Profiling of circulating cells in blood brings insights into diagnostic markers for different health and disease conditions. Differences in cellular profiles can be leveraged for personalized medicine design. Single-cell technologies has advanced our understanding of cell subpopulations and their distinct functions. While increasing evidence has shown that neutrophils and platelets are heterogeneous populations with multiple subpopulations, we are unclear about how they are distinct in biological functions and how the compositions and circadian dynamics differ between individuals. We investigate intra-individual and inter-individual heterogeneity in circulating neutrophils and platelets at a single-cell level to in different health, disease, and treatment conditions to fully understand their distinct roles in our body.
Point-of-Care Neutrophil Counter
Lead Researcher: Hyoann Choi

Neutrophil count is an important clinical indicator of immune system competency against infections. Reduced neutrophil count, termed neutropenia, is a common side effect of chemotherapy and is also manifested in patients with many other hematologic disorders. We aim to develop an inexpensive, portable, point-of-care device that enables neutropenia diagnosis using only several drops of blood. To accomplish this, we work on challenges with neutrophil enrichment, low-resource setting neutrophil quantification, and device designs.
Microfluidic Multi-Drug Functional Profiling Towards Personalized Clinical Predictions in Pediatric Leukemia
Lead Researcher: Kendall Williams

Treatment of pediatric T-cell acute lymphoblastic leukemia (ALL) has seen dramatic improvements over the last several decades, leading to overall survival rates of over 85%. However, for patients with relapsed/refractory disease, achieving complete remission remains extremely challenging. Improving treatment outcomes for these patients will require increasingly personalized treatment strategies that make use of functional screens in addition to molecular profiling technologies. Given the past success of combination chemotherapy in cancer treatment and recent promise for newly developed targeted agents, functional assays that are able to evaluate patient response to combinations of therapies will likely be most effective in identifying clinically actionable treatment protocols. Moreover, those that can achieve this with minimal patient sample and reduced experimental workload will have the greatest chance of clinical translation and integration into clinical workflows. To that end, this work aims to develop a microfluidic assay to directly test candidate combinations of therapeutics in patient derived T-ALL cells. This will allow for analysis of response to combinations of three candidate drugs while minimizing required patient sample. Once validated, this assay can be used to identify biomarkers of response to novel combination regimens in pediatric T-ALL, and ultimately, could be used to prospectively guide therapy in newly diagnosed patients.
Significant differences in single-platelet biophysics exist across species but attenuate during clot formation

Lead researcher: Oluwamayokun “Mo” Oshinowo
Human, canine, ovine, and porcine platelets exhibit disparate biophysical signatures, whereas human and murine platelets are similar. Multiple biophysical parameters integrate during clot formation, measured by bulk clot contraction, and attenuate biophysical differences.
A microengineered vascularized bleeding model that integrates the principal components of hemostasis
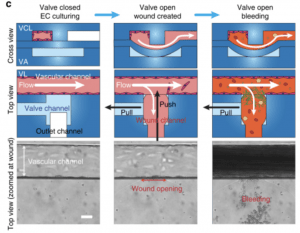
Lead researchers: Yumiko Sakurai, MS, Elaissa Hardy, PhD, and Byungwook Ahn, PhD
Hemostasis encompasses an ensemble of interactions among platelets, coagulation factors, blood cells, endothelium, and hemodynamic forces, but current assays assess only isolated aspects of this complex process. Accordingly, here we develop a comprehensive in vitro mechanical injury bleeding model comprising an “endothelialized” microfluidic system coupled with a microengineered pneumatic valve that induces a vascular “injury”. With perfusion of whole blood, hemostatic plug formation is visualized and “in vitro bleeding time” is measured. We investigate the interaction of different components of hemostasis, gaining insight into several unresolved hematologic issues. Specifically, we visualize and quantitatively demonstrate: the effect of anti-platelet agent on clot contraction and hemostatic plug formation, that von Willebrand factor is essential for hemostasis at high shear, that hemophilia A blood confers unstable hemostatic plug formation and altered fibrin architecture, and the importance of endothelial phosphatidylserine in hemostasis. These results establish the versatility and clinical utility of our microfluidic bleeding model.
Microvasculature-on-a-chip for the long-term study of endothelial barrier dysfunction and microvascular obstruction in disease

Lead researcher: Yongzhi Qiu, PhD
Alterations in the mechanical properties of erythrocytes occurring in inflammatory and hematologic disorders such as sickle cell disease (SCD) and malaria often lead to increased endothelial permeability, haemolysis, and microvascular obstruction. However, the associations among these pathological phenomena remain unknown. Here, we report a perfusable, endothelialized microvasculature-on-a-chip featuring an interpenetrating-polymer-network hydrogel that recapitulates the stiffness of blood-vessel intima, basement membrane self-deposition and self-healing endothelial barrier function for longer than 1 month. The microsystem enables the real-time visualization, with high spatiotemporal resolution, of microvascular obstruction and endothelial permeability under physiological flow conditions. We found how extracellular heme, a hemolytic byproduct, induces delayed but reversible endothelial permeability in a dose-dependent manner, and demonstrate that endothelial interactions with SCD or malaria-infected erythrocytes cause reversible microchannel occlusion and increased in situ endothelial permeability. The microvasculature-on-a-chip enables mechanistic insight into the endothelial barrier dysfunction associated with SCD, malaria and other inflammatory and haematological diseases.
Interdigitated microelectronic bandage augments hemostasis and clot formation at low applied voltage in vitro and in vivo

Lead researcher: Elaissa Hardy, PhD
Hemorrhage or uncontrolled bleeding can arise either due to a medical condition or from a traumatic injury and are typically controlled with the application of a hemostatic agent. Hemostatic agents are currently derived from animal or human products, which carry risks of blood borne infections and immune dysregulation. Therefore, the need exists for novel biomedical therapies not derived from animal or human products to achieve hemostasis. Accordingly, we created an interdigitated microelectronic bandage that applies low voltage electrical stimulation to an injury site, resulting in faster clot formation without excessive heating, accelerated fibrin formation, and hemostasis overall. Our interdigitated microelectronic bandage found fibrin formed 1.5× faster in vitro. In vivo, total cessation of bleeding was 2.5× faster, resulting in 2× less blood loss. Electricity has been used in medical applications such as defibrillation, cauterization, and electrosurgery, but scant research has focused on hemostasis. Here we report a novel surface treatment using an interdigitated microelectronic device that creates rapid hemostasis in both in vitro and in vivo bleeding models with low applied voltages, representing a new and novel class of hemostatic agents that are electrically-based.
Microfluidic Transduction Harnesses Mass Transport Principles to Enhance Gene Transfer Efficiency

Lead researcher: Reginald Tran, PhD
Ex vivo gene therapy using lentiviral vectors (LVs) is a proven approach to treat and potentially cure many hematologic disorders and malignancies but remains stymied by cumbersome, cost-prohibitive, and scale-limited production processes that cannot meet the demands of current clinical protocols for widespread clinical utilization. However, limitations in LV manufacture coupled with inefficient transduction protocols requiring significant excess amounts of vector currently limit widespread implementation. Herein, we describe a microfluidic, mass transport-based approach that overcomes the diffusion limitations of current transduction platforms to enhance LV gene transfer kinetics and efficiency. This novel ex vivo LV transduction platform is flexible in design, easy to use, scalable, and compatible with standard cell transduction reagents and LV preparations. Using hematopoietic cell lines, primary human T cells, primary hematopoietic stem and progenitor cells (HSPCs) of both murine (Sca-1+) and human (CD34+) origin, microfluidic transduction using clinically processed LVs occurs up to 5-fold faster and requires as little as one-twentieth of LV. As an in vivo validation of the microfluidic-based transduction technology, HSPC gene therapy was performed in hemophilia A mice using limiting amounts of LV. Compared to the standard static well-based transduction protocols, only animals transplanted with microfluidic-transduced cells displayed clotting levels restored to normal.
Cellular softening mediates leukocyte demargination and trafficking, thereby increasing clinical blood counts
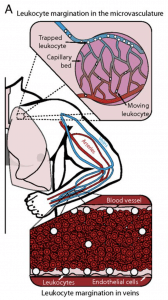
Lead researchers: Meredith Fay, PhD and David Myers, PhD
Leukocytes normally marginate toward the vascular wall in large vessels and within the microvasculature. Reversal of this process, leukocyte demargination, leads to substantial increases in the clinical white blood cell and granulocyte count and is a well-documented effect of glucocorticoid and catecholamine hormones, although the underlying mechanisms remain unclear. Here we show that alterations in granulocyte mechanical properties are the driving force behind glucocorticoid- and catecholamine-induced demargination. First, we found that the proportions of granulocytes from healthy human subjects that traversed and demarginated from microfluidic models of capillary beds and veins, respectively, increased after the subjects ingested glucocorticoids. Also, we show that glucocorticoid and catecholamine exposure reorganizes cellular cortical actin, significantly reducing granulocyte stiffness, as measured with atomic force microscopy. Furthermore, using simple kinetic theory computational modeling, we found that this reduction in stiffness alone is sufficient to cause granulocyte demargination. Taken together, our findings reveal a biomechanical answer to an old hematologic question regarding how glucocorticoids and catecholamines cause leukocyte demargination. In addition, in a broader sense, we have discovered a temporally and energetically efficient mechanism in which the innate immune system can simply alter leukocyte stiffness to fine tune margination/demargination and therefore leukocyte trafficking in general. These observations have broad clinically relevant implications for the inflammatory process overall as well as hematopoietic stem cell mobilization and homing.